Environment & Energy
Related: About this forumSome Insight Into the Energy Costs Associated with Direct Air Capture of Carbon Dioxide.
The paper to which I will briefly refer in this post is this one: Exploring Geometric Properties and Cycle Design in Packed Bed and Monolith Contactors Using Temperature-Vacuum Swing Adsorption Modeling for Direct Air Capture, Valentina Stampi-Bombelli and Marco Mazzotti, Industrial & Engineering Chemistry Research 2024 63 (45), 19728-19743.
It is - often rightly - remarked that the concept of direct air capture, (DAC) is a means, like hyping "hydrogen" to rebrand fossil fuels as "green," is a potentially a pathway to greenwashing fossil fuels. This argument has some merit. This said, because we have left future generations with a destroyed planetary atmosphere, the research is, to my mind, worthy, since, depending on the resources available to them - which are diminishing in our orgy of consumerism coupled with wishful thinking - they may wish to attempt restoration of the world that once was, should the concept of "history" survive, which it may not do in Orwellian times.
The problem is that to capture CO2 from the air, or for that matter, flue gas, requires energy, significant quantities of it. The more dilute the CO2 is, the more energy is required to overcome the entropy of mixing. In addition, if one wishes to put the CO2 to use, rather than to propose vast unsustainable CO2 dumps, which are in fact, like hydrogen, an approach to greenwashing dangerous fossil fuels.
I have my own ideas about DAC, which involve process intensification using air based Brayton cycles, with both electricity and CO2 being side products, rather than the main product, by increasing exergy capture, essentially increasing the recovery of thermal energy, i.e., raising the energy efficiency in the use of nuclear generated heat.
The text refers to the 2015 Paris Agreement on what was then called "climate change" and to which I now refer as "extreme global heating," as this is what we now observe in our Godotian approach to energy, which is to wait for a so called "renewable energy" nirvana that did not come, is not here, and won't come. In Beckett's "Waiting for Godot," Vladimir and Estragon consider suicide, whereas in waiting for the so called "renewable energy" nirvana, we are actively committing suicide, slowly, by degrees, literally and figuratively, a less than minor difference. What is relevant to the issue is not the details of the paper, which is wonderful as a tool in considering the issues of gas/liquid equilibria expressed in mathematical terms, but rather some insight to the energy cost of direct air capture. This is the energy associated with overcoming the entropy of mixing by taking concentrated carbon - what the dangerous fossil fuels were before combustion and dealing with them in a dilute form, the low, but dangerously rising levels found in air.
From the text:
In alignment with the 2015 Paris Agreement, global efforts to limit warming to a 1.5 °C increase above preindustrial levels call for substantial reductions in CO2 emissions. (1) Although decarbonizing mobility, households, industry, and power generation is crucial, these measures are slow to implement and incomplete, leaving residual emissions in so-called hard-to-abate sectors that need to be addressed. Thus, negative emission technologies (NETs) that allow for carbon dioxide removal (CDR) from the atmosphere, such as afforestation, bioenergy with carbon capture and storage, and direct air capture and carbon storage, play a vital role. Direct air capture (DAC), a technology that extracts CO2 directly from the atmosphere, emerges as a promising CDR technology. Employing both solid sorbents (adsorption) and liquid solvents (absorption), DAC research and deployment are brought forward by both academia and industry, with companies like Climeworks, Global Thermostat, and Carbon Engineering leading the way. The lower energy demand for sorbent regeneration in adsorption offers an advantage over absorption (with heats of desorption of ca. 4090 kJ/mol vs 200 kJ/mol in adsorption and absorption, respectively (2,3)), yet DACs widespread adoption hinges on resolving significant technical and economic challenges.
The low concentration of CO2 and the unavoidable humidity in the air are two of the primary challenges associated with DAC. As a result, a large portion of DAC research has centered around developing materials with high CO2 capacity and selectivity over N2 and O2, (4,5) evaluating sorbent stability (4,6−8) and accurately characterizing CO2H2O coadsorption. (9−13) Amine-functionalized materials have emerged as promising sorbents, offering high CO2 capacities even in dilute CO2 conditions, often enhanced in humid environments. (12,14,15) However, despite their favorable thermodynamic properties, amine-functionalized sorbents have been shown to exhibit kinetic limitations. (11,16−20) Various studies have shown that external and internal diffusion resistances in the gas phase are the limiting mechanisms defining the adsorption kinetics, (20−22) with their limitations increasing as the feed concentration decreases. (21,22) This is particularly relevant in DAC, where a low CO2 concentration significantly affects gas-phase mass transport, contributing to slow kinetics, early breakthrough, and inefficient sorbent utilization. Experimental characterization of mass transfer kinetics under conditions relevant to DAC remains sparse despite its critical importance. (12,22,23) However, precise modeling of these dynamics is essential, as evidenced by the significant impact that the mass transfer coefficient, k, has on DAC performance through cyclic temperature-vacuum swing adsorption calculations. (24−26)
Another major challenge associated with the dilute CO2 concentration in the feed is that large volumetric amounts of air need to be processed to capture a significant amount of CO2. Coupled with the desire to operate at high air velocities to maintain short cycle times, this requirement might result in a large pressure drop across the airsolid contactor, thus increasing the energy demand of the blowers. Given that pressure drop increases with longer beds, lower bed porosities, and higher velocities, (27,28) it is intuitive to reduce bed lengths or to increase bed porosities to accommodate high air velocities. Multiple new contactor designs have been proposed to achieve the goal of reducing pressure drop while trying to maintain a compact structure. These include thin-layered packed beds arranged in compact geometries, such as radial bed contactors, (12,24−26,29−33) and structured sorbents like laminates (34) and monoliths, (2,21,23,30,31,35−40) which are compact and feature high porosities. Monoliths have gained particular attention due to their wide use in the catalyst industry and readiness at a technical level. With their parallel channel configuration, they have been shown not only to reduce pressure drop but also to enhance mass transfer kinetics in point-source CO2 capture applications, (41−46) thereby also potentially addressing the kinetic limitations inherent in DAC. Both packed bed and monolith structures present distinct advantages and drawbacks for DAC applications, each influenced by current technological and commercial realities. (28) Monoliths, although beneficial for their lower pressure drops and higher mass transfer rates, are typically constrained by lower sorbent loadings, while at the same time, monoliths tailored for DAC are not readily commercially available. On the other hand, packed beds exhibit a higher pressure drop but benefit from high sorbent loadings and the availability of commercially viable pelletized sorbents like Lewatit VP OC 1065 for easier scalability...
What is interesting is the sentence I have bolded, which gives the energy cost of two approaches, absorption and adsorption, to regeneration.
A mole of carbon dioxide (its molecular weight) is 44.0095 grams. This means to regenerate the adsorptive agent, it will take a minimum of 40 kJ of energy to regenerate an adsorptive agent to capture 44.01 grams of CO2.
Ignoring land use changes, it appears that emissions from the combustion of dangerous fossil fuels, as of 2023 is on the order of 37.8 billion tons per year.
Breakdown of Carbon Emissions by Source; 2003-2023
This translates to 3.78 X 1016 grams of CO2 which further translates to 8.59 X 1014moles of CO2.
This means that for the low end of adsorptive cost 40 kJ per mole, the energy requirement of carbon free energy would be roughly 34.4 Exajoules (EJ) of energy. This is slightly more than twice the 16 Exajoules that our multitrillion dollar combined solar and wind energy produced in 2023 for all purposes, this on a planet that was consuming 642 EJ in that year.
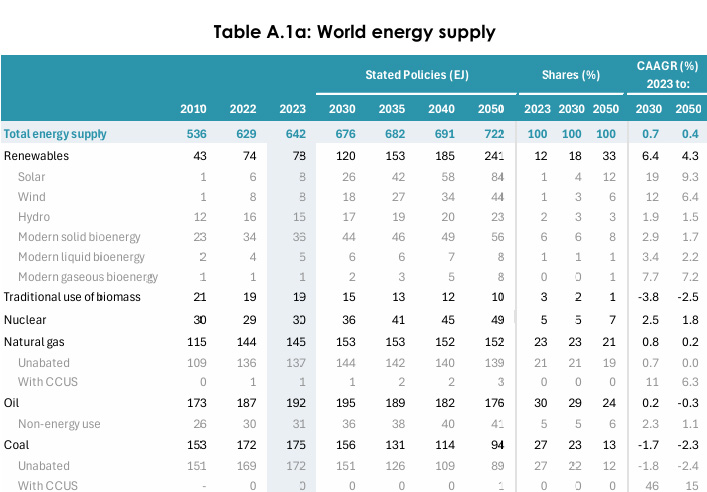
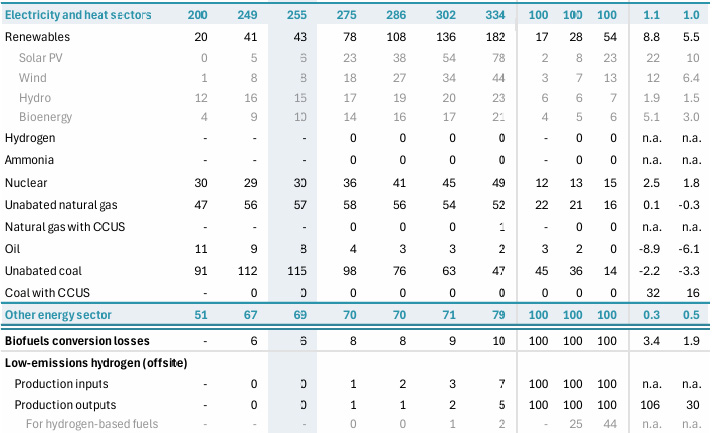
IEA World Energy Outlook 2024
Table A.1a: World energy supply Page 296.
At the upper limit for adsorptive processes, 90 kJ/mole, the energy requirement would be 77.3 EJ.
For the absorptive process, at 200 kJ/mole, the energy cost would be 171 EJ.
In the "percent talk" used to justify the useless solar and wind industries, at 40 kJ/mole, 90 kJ/mole, and 200 kJ, these energy costs are respectively 5.3%, 12.0%, and 26.8% of the current world energy supply, not to do any useful work, merely to remove a year's worth of carbon dioxide.
Note that this is simply to capture carbon dioxide from the air - that is to over overcome the entropy of mixing, not to reduce it to a liquid or solid form, which will require reproducing all of the energy, and then some (for the entropy of combustion/reduction) that put it there in the first place, nor to compress it for the putative fantasy of building huge geological carbon dioxide dumps that will inevitably leak but, like the hydrogen fantasy, is pure fossil fuel greenwashing bullshit.
It is feasible, to my mind, to recover more energy from nuclear fuels than is currently captured in the major usage thus far, which is simply to generate thermodynamically degraded electricity. But the technology for doing this is "pie-in-the-sky" as of now. We're in deep shit. I don't really weep for us on this score. We clearly never gave a shit. I do weep, instead, for future generations.
History will not forgive us, nor should it.
Happy New Year.

erronis
(17,275 posts)Wishful thinking and perpetual motion machines live on in some dreamland.
NNadir
(34,881 posts)It would be extremely difficult, and no doubt extremely expensive to build, but perhaps not to run once built.
Of course, we do not live in a wise world, so there's that. And of course, our bourgeois airheads here and elsewhere, are cheapskates, completely willing to dump the cost of their ignorance on future generations to save a dime. They prance around insipidly all the time issuing delusional statements that so called "renewable energy" - affection for which is accelerating the extreme global heating we now experience - is "cheap." (They seem not to notice that a large number of European countries want to cut off that coal burning "renewable energy" "nirvana" Germany for driving European electricity prices to unprecedented heights during episodes of Dunkelflaute, the new German word that has become an international term of disgust with antinuke stupidity.)
The basis for my perhaps over optimistic belief that a closed carbon cycle is feasible contains an admittedly dubious "appeal to authority" argument, reference to a paper by the late Nobel Laureate George Olah, who, at least was an expert in carbon chemistry, meaning that the "authority" is somewhat more real than some fossil fuel greenwashing airhead announcing that because Energy Secretary Jennifer Granholm thinks a hydrogen economy is viable, it therefore is. (Secretary Granholm is an outstanding woman, but she is a politician, not a scientist.)
The paper in question is this one: Anthropogenic Chemical Carbon Cycle for a Sustainable Future George A. Olah, G. K. Surya Prakash, and Alain Goeppert Journal of the American Chemical Society 2011 133 (33), 12881-12898
I reference this paper often.
To see a possible route achieving this goal, one should consider the exergy recovery of nuclear reactors which is, to my way of thinking, unacceptably low, as they are designed to be (largely) Rankine type steam cycle devices designed to dissipate, rather than use, waste heat.
According to the World Nuclear Association, in 2023, the world produced 9% of the world's electricity, which amounted to 2,601,617 GWh of electricity, or 9.37 Exajoules (EJ). In the OP, by reference to the IEA data, the primary energy associated with nuclear power was 30 EJ, meaning, to carry one insignificant figure, that the thermal efficiency of nuclear power plants on the planet was 31.2%. This also means that 20.6EJ of heat was rejected to the environment, only a small trivial quantity of which was utilized for space heating in urban networks.
It would be energetically expensive to recover this heat for existing reactors with heat pumps, further reducing the thermal efficiency (for the purpose of generating electricity) but suppose instead we built reactors that were designed to not dissipate heat but to let it flow in concentrated form through a series of heat exchangers designed to extract exergy from that heat, that is process intensification. The laws of thermodynamics limit how much of that heat might be captured, but crude calculations on my part, suggest that it might be possible to approach 80% thermal efficiency, rather than 31% efficiency.
An air driven Brayton cycle would necessarily give an avenue to process air, to pressurize it. In this process, CO2 capture might be achieved as a side product of the process, albeit at an energy penalty for the overall efficiency of the system, but utilizing energy that is currently rejected to the environment.
Suppose we generated 500 EJ of primary energy each year from nuclear energy, requiring 6,000 tons of plutonium per year, roughly, and instead of 31% efficiency, achieved, let's say 70% efficiency, with not all of the exergy coming as electricity, but perhaps in the form of chemical energy (as Olah's paper suggests, DME or Methanol). This would provide about 200 EJ of recovered energy that would be put to use, not rejected. Under these conditions, air capture borders on feasible. I note that it is less energetically intensive to recover CO2 from seawater than from air, but that's another story.
These things lead me to suspect feasibility, which is nowhere near the same as saying "likely." At the end of my life, I'm sure the embrace of stupidity is far more likely than the embrace of wisdom.
Thanks for your comment.