Science
Related: About this forumThe Genetics of the Heavy Metal Resistome of Microorganisms.
I like to approach accessible subjects about which I know nothing or very little. I have been required on a very superficial level on genomics, although it is unlikely I will ever become expert in the area; there won't be time.
I am aware however of the biochemistry of heavy metals, in particular with respect to their role in catalysis and transport, and I work regularly with proteomics and I am very aware of heavy metal pollution driven by coal combustion and other sources.
In this regard, I came across a cool paper today in the recent issue of Environmental Science and Technology, this one: MRG Chip: A High-Throughput qPCR-Based Tool for Assessment of the Heavy Metal(loid) Resistome, Jiaojiao Zhu, Qiong Huang, Xinyi Peng, Xinyuan Zhou, Shenghan Gao, Yuanping Li, Xuesong Luo, Yi Zhao, Christopher Rensing, Jianqiang Su, Peng Cai, Yurong Liu, Wenli Chen, Xiuli Hao, and Qiaoyun Huang Environmental Science & Technology 2022 56 (15), 10656-10667
Regrettably I'm not going to have time to discuss the paper in any detail, but the introductory paragraphs certainly define the flavor:
Microorganisms play an irreplaceable role in the biogeochemical cycling of metals. (14−17) Metals, such as Cu, Zn, cobalt (Co), and nickel (Ni), are essential micronutrients for microbial metabolism. These metals function as cofactors, involved in central bioenergetic and biogeochemical processes (e.g., respiration, photosynthesis, antioxidant defense, and nitrogen cycling). (18−20) However, due to the high affinity to thiol groups and/or oxidation properties, metals can be toxic and target multiple cellular processes through attacking ironsulfur (FeS) clusters, replacing essential metals, dysfunction of proteins, oxidation of lipid and nucleic acid, and interfering nutrient assimilation. (1,18) To balance the metabolic function demand and metal toxicity, microbes have evolved sophisticated homeostatic mechanisms to maintain essential metals at a sufficient level. (19) These mechanisms are categorized as efflux, transformation, sequestration, precipitation, and reduced uptake. Among these, efflux pumps are the most expedient mechanism for bacteria to cope with excess metal ions, which is mainly mediated by three types of transport systems: the P-type ATPase, the Cation Diffusion Facilitator (CDF) efflux transporter, and the multicomponent Resistance-Nodulation-Division (RND) transport system. (21) Enzyme-mediated metal biotransformations alter metal speciation through redox and (de)methylation. (18,22,23) Metallothionein or chaperone proteins sequester intracellular metal ions to alleviate toxicity. (24) For instance, Cu chaperone CopZ can bind excess cytoplasmic Cu in Enterococcus hirae, (23) and metallothionein SmtA confers Cd resistance in Cyanobacteria. (25) In addition, various high- and low-affinity uptake systems contribute to the import of essential metals and are of importance for bacterial metal homeostasis. (26) These mechanisms protect bacteria from metal toxicity and meanwhile drive the metal biogeochemical cycling.
To date, the genetic basis for bacterial metal resistance and related biochemical pathways have been elaborated in considerable detail by pure culture-based studies. (27−29) However, the resistance systems identified in several isolates could not provide comprehensive insights into the scenario of resistance strategies in complex environments, since only a tiny fraction of microbes is culturable. Up to now, profiling the metal resistance determinants at the community level is still a great challenge, partially due to lack of comprehensive and targeted tools for gene quantification. Although metagenomic and metatranscriptomic approaches have been used to identify the gene profile underlying microbial resistance to metals, (30−33) they are still facing the challenge of rapid detection and quantification. (34,35) HT-qPCR based quantification approaches overcome the obstacle of meta-omic approaches for cost-effective and accurate quantification, which has been widely used to describe and interpret the microbial functional structure in various ecosystems. (36−40) However, the primer sets which are currently available for detecting metal resistance determinants are restricted to specific types of metals, resistance mechanisms, and bacterial species. (5) Some primer sets target sequences with a length of over 500 bp, which are too long and not suitable for the qPCR assay. Thus, the lack of reliable primer sets hinders the assessment of the metal resistome and microbially mediated metal biogeochemical cycling.
Here, a novel HT-qPCR-based chip, termed the MRG chip, was developed for the comprehensive profiling of known prokaryotic metal resistance genes (MRGs) involved in the homeostasis of 9 metals...
Here's the introductory cartoon:
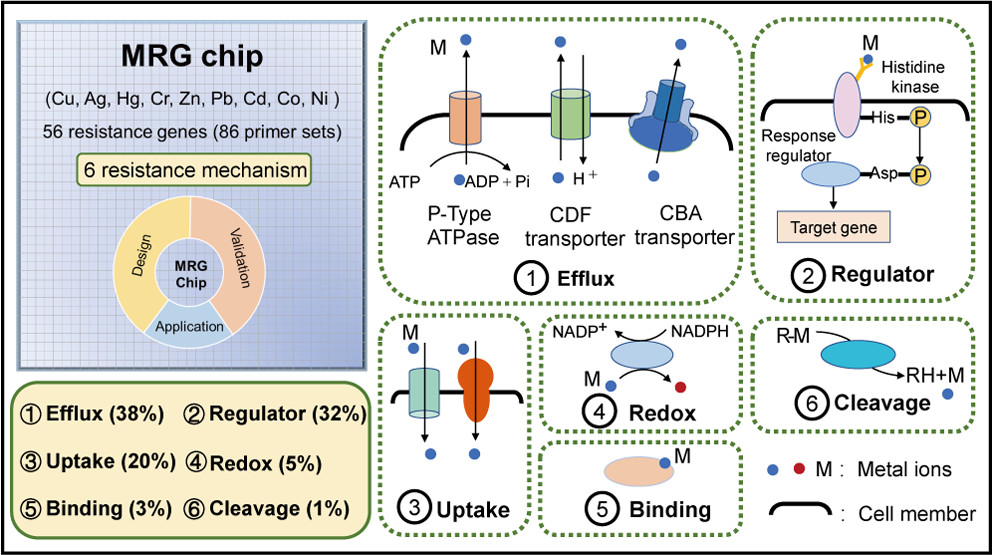
A figure from the paper giving insight to the overall approach of the research:
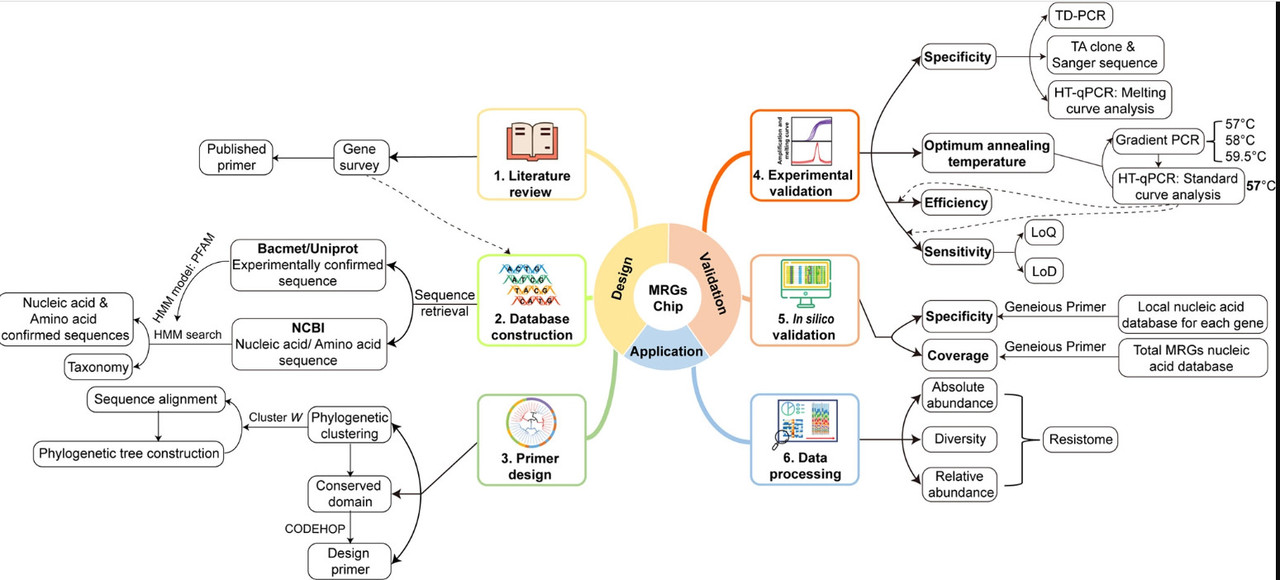
The caption:
Figure 1. Flowchart of major steps for the development of the MRG chip, including literature review, database construction, primer design, experimental validation, in silico validation, and data processing.
Understanding the genomics of metal processing in organisms can have profound effects in the future for environmental remediation and indeed for the recovery of metals. (I have been aware of certain uranium complexing proteins in some coral species, but this work is far more general.)
Cool, I think.

3catwoman3
(25,439 posts)en suite bathroom were anti-microbial.
NNadir
(34,661 posts)...in low concentrations, but toxic in high concentrations, as is the case with other elements, notably selenium.